The spike protein is a protein on the surface of the SARS-CoV-2 virus. The COVID-19 vaccines work by getting cells to produce this protein, with some modifications,1 so that the body will generate neutralizing antibodies to it.
Multiple studies have shown that spike protein is harmful, but there’s a lot we don’t know about how it causes harm.
This article will attempt to answer a seemingly simple question: does the spike protein get into the nucleus of cells?2
According to vaccine manufacturers, the answer is no. Unfortunately, we’ll see that this question is not straightforward to answer. However, it can teach us a lot about how to interpret studies.
Background: the nucleus
The nucleus houses the DNA of the cell in most3 eukaryotic cells.
It’s wrapped in a double membrane called the nuclear envelope. Only certain things are allowed in and out of nucleus.
Small molecules can move freely through small holes in the nuclear envelope called nuclear pores, but bigger molecules need the help of special carrier proteins, or importins.
What goes in and out of the nucleus is tightly regulated. Obviously we don’t want foreign proteins, especially cytotoxic ones, getting into the nucleus and potentially creating havoc, like damage to DNA or DNA repair mechanisms.
Where would we expect to see spike protein in cells after vaccination?
According to the vaccine manufacturers, spike protein generated from the vaccines was not intended to get into the nucleus of cells.
Here’s what’s supposed to happen, according to a Moderna (Spikevax) report (p.60):
The delivered mRNA does not enter the cellular nucleus or interact with the genome, is non-replicating, and is expressed transiently4 mainly by dendritic cells and subcapsular sinus macrophages.
As for the Comirnaty (Pfizer, BioNTech) vaccine, according to their report (p.13):
The mRNA is translated into the SARS-CoV-2 S protein in the host cell cytosol. The S protein is then expressed on the cell surface where it induces an adaptive immune response.
And in this report they say (page 8):
The expressed S protein co-localised with an endoplasmic reticulum (ER) marker, suggesting the S protein is synthesized and processed within the ER for surface expression or secretion.
So theoretically, it’s supposed to look like this:
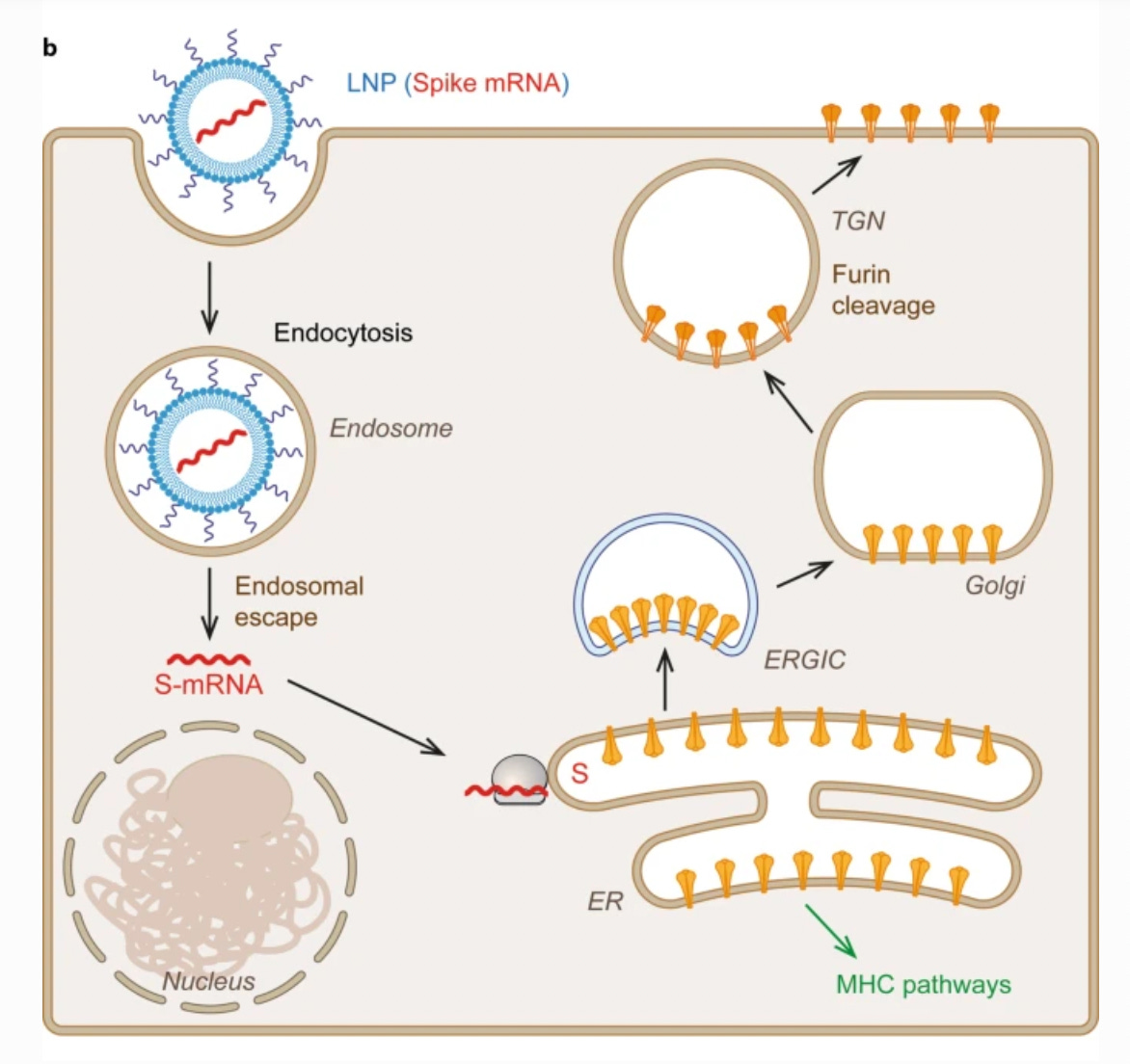
In the above diagram, the lipid nanoparticles, or LNPs, house the mRNA (red), which encodes for spike protein. Once inside the cell, the mRNA gets translated by ribosomes. This image just shows one example of a ribosome; it looks like a beige hamburger.
The spike proteins are supposed to be synthesized by ribosomes associated with the ER (endoplasmic reticulum), which is a network of interconnected sacs where protein biosynthesis and modification occurs.
The ER extends throughout the cytoplasm, but is closely associated with the nucleus, and continuous with the nuclear envelope:
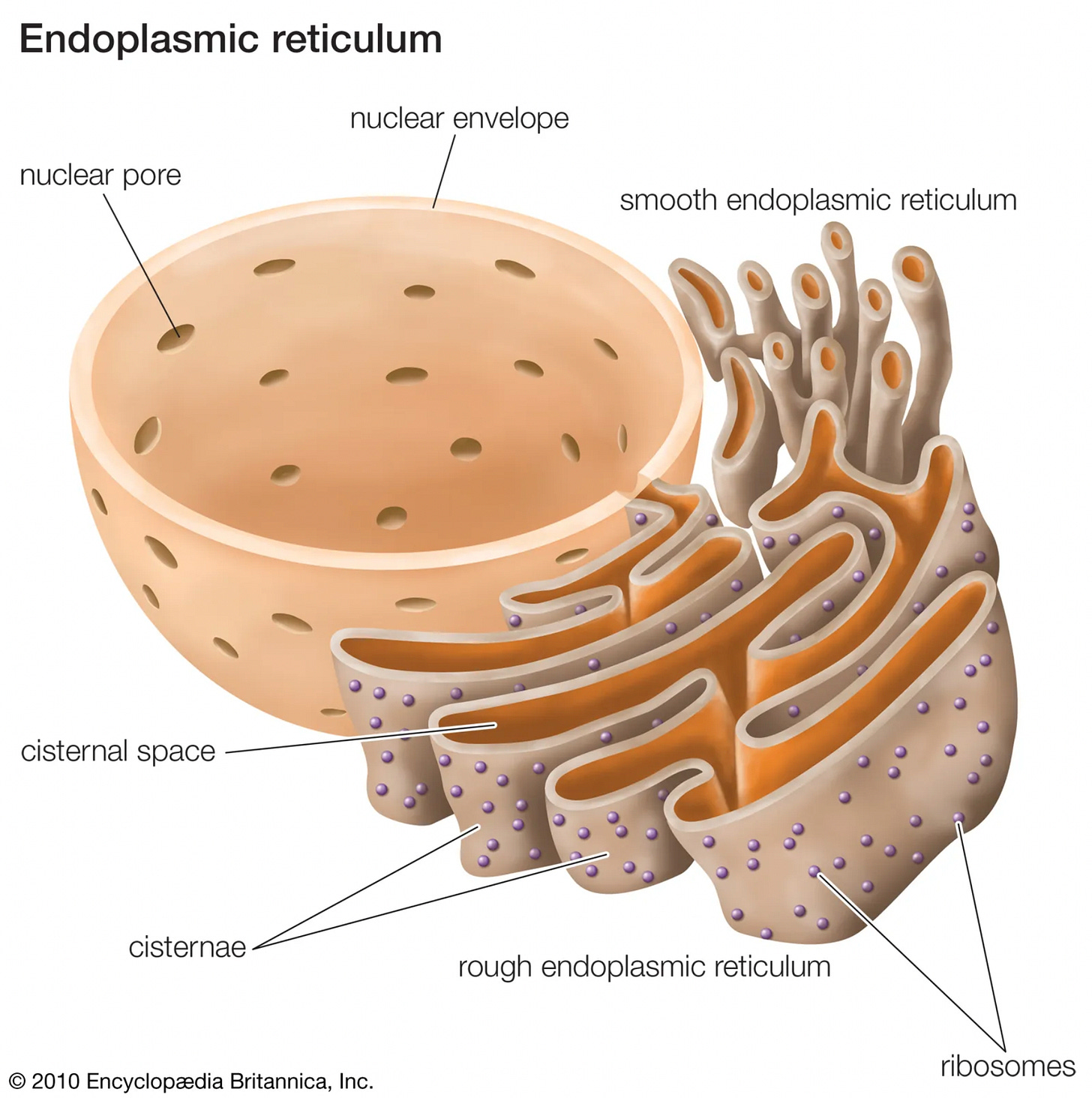
Finally, the spike proteins are supposed to end up on the surface of the cells, where they can be “presented” to immune cells.
Summary of the results on spike protein localization
So is the spike protein only found in the cytosol or ER or on the surface of cells, as vaccine manufacturers claim?
This table summarizes the results of all the studies I could find where any type of human cell was exposed to spike protein or spike subunits, and subcellular localization (the location within cells) of spike was investigated:
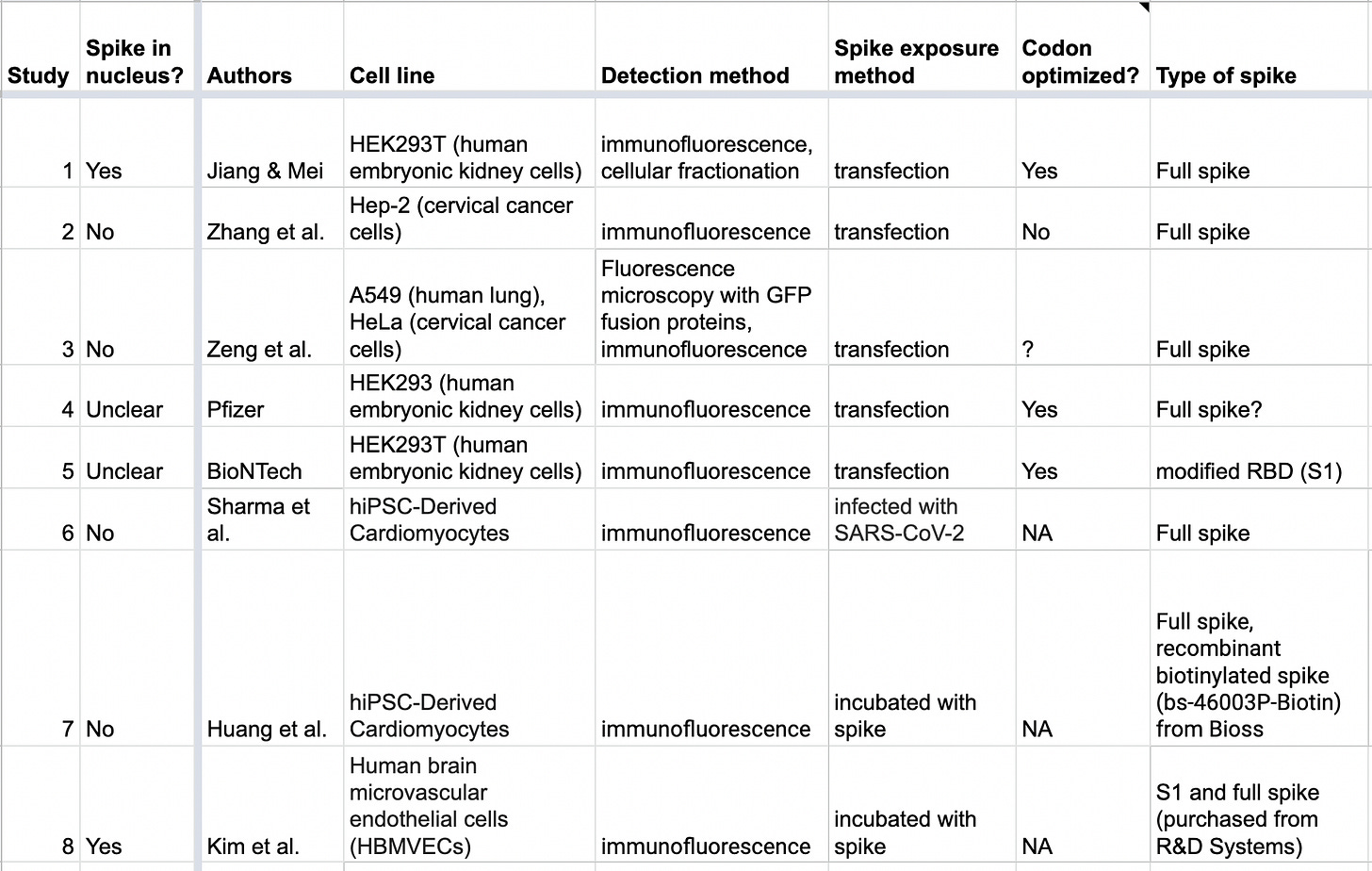
The second column indicates whether the study found spike protein getting into the nucleus or not. As you can see, the results are conflicting.
Here’s a quick summary of what each study found:
Study 1
This paper5 got a lot of attention when it came out:
SARS–CoV–2 Spike Impairs DNA Damage Repair and Inhibits V(D)J Recombination In Vitro
Each column below shows cells expressing a different SARS-CoV-2 protein (Nsp1, Nsp5, Nsp9, etc.).
The top row shows cells stained with a blue stain, DAPI. Wherever you see a blue oval, you’re basically looking at a nucleus. The green in the second row is supposed to show the expressed SARS-CoV-2 proteins.
You’ll see that all the viral proteins (green) got into the nucleus in all cases, with the exception of the nucleocapsid protein (circled in red).
Study 2
In this study by Zhang et al., just like in the first study, they observed NSP1, NSP5, NSP9 and NSP13 getting into the nucleus. However, they did not find spike protein in the nucleus.
In the image below, spike is in green, and the DNA is stained blue:
The image below shows what it’s supposed to look like when a protein, in this case NSP13, is in the nucleus:
There, you’ll see green overlapping with blue.
Study 3
This study reported that spike protein “distributed in the cytoplasm… although a weak fluorescence signal was observed in the plasma membrane of some cells” (Fig. 5).
Study 4
Earlier I had mentioned a report for the Comirnaty vaccine. That report contains data on subcellular localization. In the below image, the blue is DNA, red is the ER and Golgi, while they say green is S1.
What’s strange is that the BNT162b2 vaccine is supposed to encode for full length spike protein, not just S1, which is a subunit of spike, so I’m not sure why it says “S1” in this image.
But putting that aside, on page 35 it says:
Co-localisation of the S-protein antigen with an ER marker was detected, suggesting the S protein is processed within the ER.
It looks like the green is mostly where the ER is supposed to be, but it also looks like there’s some spike in the nucleus as well. It’s hard to tell.
That’s why I marked these results as “unclear.”
Study 5
In an “investigator’s brochure” for BioNTech vaccines, they look at several vaccine candidates. Page 25 contains something very interesting:
The first row shows non-transfected control cells.
The second row shows the result of transfecting cells with “modRNA V5” which encodes for a variant of SARS-CoV-2 RBD. “RBD” stands for the receptor binding domain, a part of the S1 subunit of spike.
It looks like this localizes to the ER, though there might be some overlap with the nucleus as well. Again, let’s say these results are “unclear.”
You’ll notice that the third row was blacked out/redacted. My guess is that it had results of V8 or V9 based on what was in the introductory text of this section, which I've highlighted in yellow.
It’s unfortunate that we don’t have these results, because V8 and V9 were two variants of the BNT162b2 vaccine.6 Presumably one of these led to the approved BioNTech-Pfizer vaccines.
I can’t help but wonder whether the results were redacted because they showed lots of spike protein in the nucleus.
Study 6
This study by Sharma et al. found spike (Fig. 1D) “localized at perinuclear regions,” which means “around or surrounding the nucleus.” This is what you’d expect if it was localized to the endoplasmic reticulum.
Study 7
In this study by Huang et al., they also found spike “around the nucleus.” The red is supposed to be spike protein and blue is DNA stained with DAPI:
Study 8
In this study by Kim et al., they found both S1 and full spike in the nucleus.
Why the conflicting results?
What explains the conflicting results?
Maybe it was because the cells used in the studies were derived from different tissues (cell lines). Moreover some studies used full length spike while others used just a piece of spike.7
The studies also used different ways to expose cells to spike. The methods were:
Transfection, where they inserted DNA or mRNA into cells to get them to make spike, as what happens under COVID-19 vaccination
Infection with SARS-CoV-2
Incubation with spike
The first five studies used transfection:
They were probably most similar to what happens under COVID vaccination.
The COVID mRNA vaccines also used codon optimization, which is supposed to improve gene expression and protein production, so you could argue that the studies that did this were most similar to what happens under COVID vaccination.8
However, the studies that incubated cells with spike are also relevant to what happens under vaccination, since we know that spike, or subunits of spike, can float around in the body after vaccination9 and potentially get into cells.
The studies also used different ways to detect subcellular localization.
This turns out to matter a lot. In fact, there’s a whole body of literature on how tricky it is to determine subcellular localization.
Some researchers might even claim that the results of all or most of these studies could be artifacts.
What are artifacts?
An artifact is something that is observed that is not naturally present but occurs as a result of experimental procedure.
For example, suppose you want to compare tissue samples that were collected from people of two different countries; one where there are a lot of fat people and one where most people are skinny.
But let’s say that unbeknownst to you, the samples from the fat country were transported over longer distances and often mixed more vigorously or even contaminated with bacteria as a result.
When you look at the samples from the fat country, you might see more of this kind of thing:
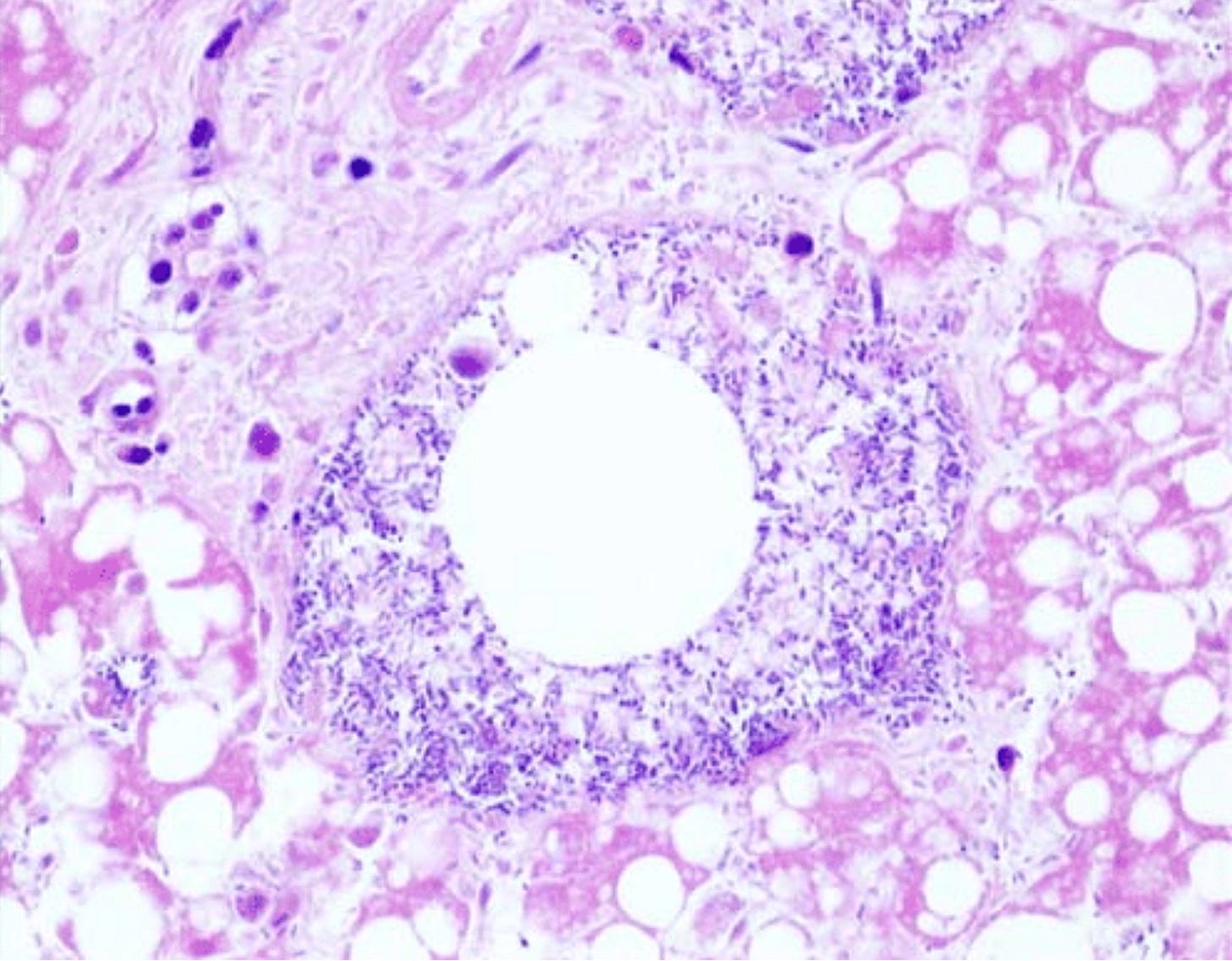
The round empty space in the middle is an air bubble surrounded by bacteria, but you might erroneously conclude that it’s the result of some pathology linked to obesity, instead of a result of those samples having been transported for longer distances and more bubbly10 and contaminated as a result.
This is an example of arriving at a conclusion based on an artifact.
Artifacts in immunofluorescence
Every spike study discussed here used a variation of a method called “immunofluorescence,” which is also sometimes called “immunolabeling.”
This method usually includes a “fixation” step, which kills the cells and supposedly “freezes” all the cellular components in place, and a “permeabilization” step, which allows antibodies to get into the cells and organelles (the antibodies allow us to visualize our proteins of interest).
Unfortunately, there’s a whole body of literature that warns about artifacts arising from immunofluorescence/immunolabeling.
A 2012 review published in Nature Methods by Schnell at al., called Immunolabeling artifacts and the need for live-cell imaging, points out that artifacts arising from immunofluorescence studies are an “often under-recognized problem”:
The results of protein-localization experiments in cells and tissues can differ drastically depending on how fixation and permeabilization are performed and even on cell type.
That review goes through several cases where different fixation and permeabilization methods lead to different results. These methods, which are always looking at dead cells, also lead to different results from methods using live cells. Schnell et al. argue that immunofluorescence studies should be complemented with studies using living cells, though those aren’t perfect either.11
The authors of this review argue that “no single piece of evidence is sufficient to address the issue,” though “all approaches can reveal useful information.”
A study from 1992 by Melan et al., Redistribution and differential extraction of soluble proteins in permeabilized cultured cells, tested several different protocols and came to similar conclusions:
These results show that misleading apparent localizations of soluble proteins can result from their redistribution and/or differential extraction during the preparation of cells for primary antibody application.
From a paper from 2009, Exploring the Function-Location Nexus: Using Multiple Lines of Evidence in Defining the Subcellular Location of Plant Proteins:
The compilation of protein locations from a variety of studies using different methods readily reveals apparent conflicts or discrepancies in location attribution by different researchers.
Here’s part of a table from their paper comparing some of the methods used to determine localization of several proteins:
In particular, look at what’s highlighted in yellow and compare “in vivo distributions” with “Fixation before extraction,” which is what most of the spike studies did. If you look at the rows for BSA and LDH proteins, you’ll see that the in vivo method did not show them going into the nucleus whereas the “Fixation before extraction” method did.
In this paper, Is VP22 nuclear homing an artifact?, they looked at a herpes simplex virus protein called VP22 and concluded that previously reported nuclear localization of this protein was an artifact of fixation.12
Here are some more papers on this topic:
This is just the tip of the iceberg. Unfortunately, many researchers who use immunofluorescence may not be aware of this body of literature.
Why is this method prone to producing artifacts?
What is it about immunofluorescence that makes it prone to yielding artifacts?
Let’s quickly look back at Study 1. Recall that in that study they got cells to express various SARS-CoV-2 proteins. Each protein was also expressed with something called a His-tag attached; this tag allows us to visualize the viral protein of interest by doing the following:
First we get “primary antibodies” to bind to the His-tags that are attached to the viral proteins of interest.
Then we get secondary antibodies to attach to the primary antibodies. These secondary antibodies can fluoresce green. So wherever we see fluorescent green in a cell, we are looking at a proxy for the viral protein of interest.
Here’s how we actually get the primary and secondary antibodies to do what we want (protocol taken from Study 1):
Translation: they took the cells that expressed the viral proteins of interest, and “fixed” them with paraformaldehyde; this step is supposed to “freeze” all the cellular components in place.
They then added something called Triton X-100, which is a surfactant that can permeabilize membranes.
We do this so the primary and secondary antibodies can reach the viral proteins of interest. If those proteins of interest are in the nucleus, the primary and secondary antibodies need to have access to the nucleus too.
You might see where we could get some problems here: the fixation step might not truly freeze all the cellular components in place, and the permeabilization step might actually allow the viral proteins to get into places where they otherwise might not have, like in the nucleus.
All the spike studies used different protocols
Every spike study13 discussed in this article had variations in how they fixed or permeabilized their cells.
For example, here is the protocol from Study 1 again:
And here is the protocol that Study 2 used:
Did the slightly higher concentration of surfactant (Triton X-100) in the first protocol lead to more permeabilization of the nuclear membrane, resulting in spike protein getting into the nucleus where it otherwise might not have? Did they do that step at room temperature, which might lead to more permeabilization than doing it on ice, like the second protocol did?
If you’re thinking that all these variations in protocols were there for good reasons, you’re probably giving researchers too much credit.14
It might be that some of the variations don’t matter much, but we don’t know, because no one has done a comprehensive experiment to see what we get with all these variations in protocols, with these particular cell lines, with this particular protein.
However, our default assumption should be that it does matter, given the body of literature showing that it has, in at least some cases.
What did the other methods show?
There were two spike studies that also used some non-immunofluorescence methods to determine spike protein localization; could those tell us anything useful?
Using cellular fractionation
In Study 1, in addition to immunofluorescence they also did something called cellular fractionation, which separates out different components of the cell using multiple rounds of centrifugation.
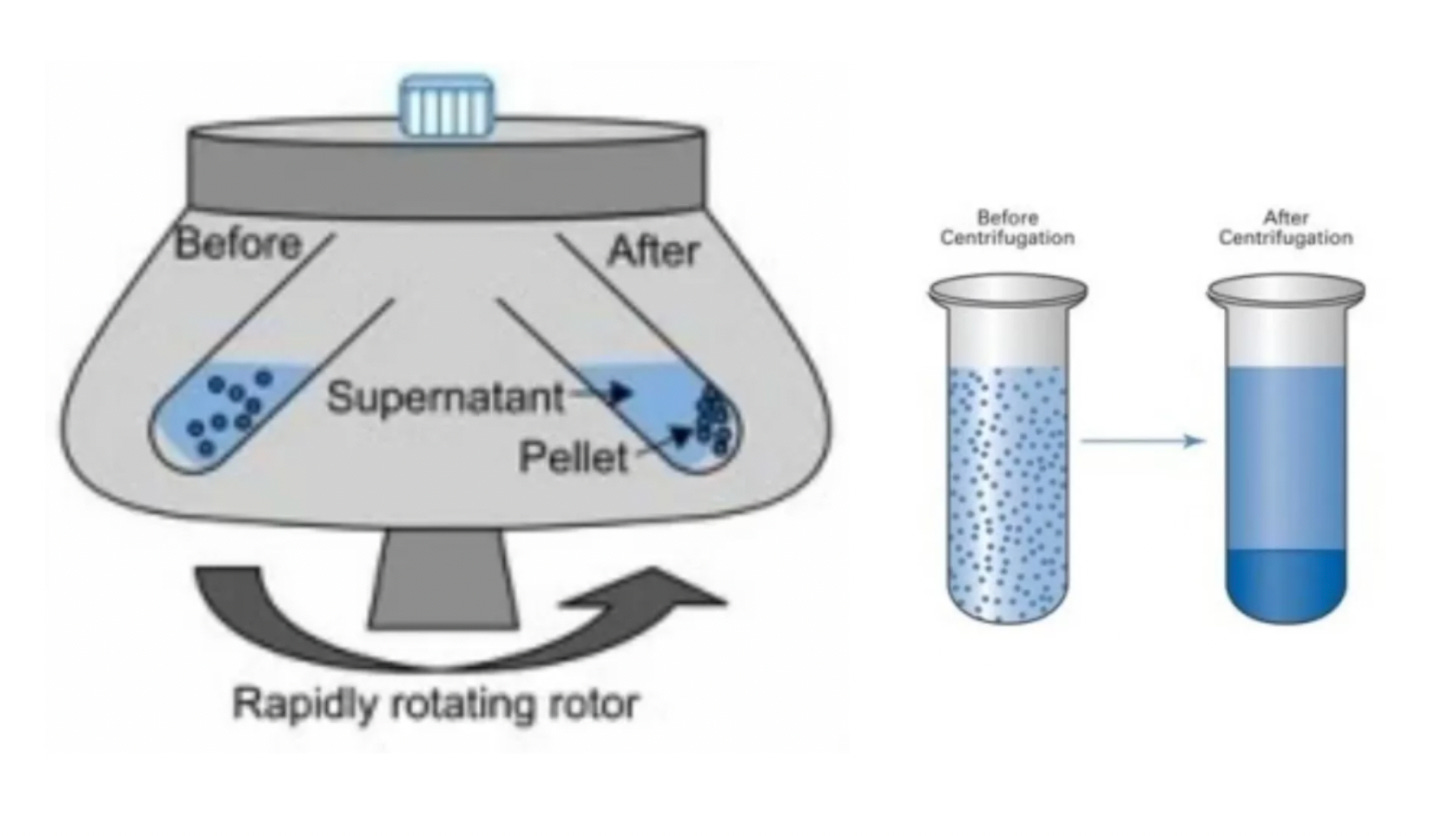
The results show that much of the spike is in the “membrane fraction,” which includes the ER, but quite a bit of spike was also in the nuclear fractions:
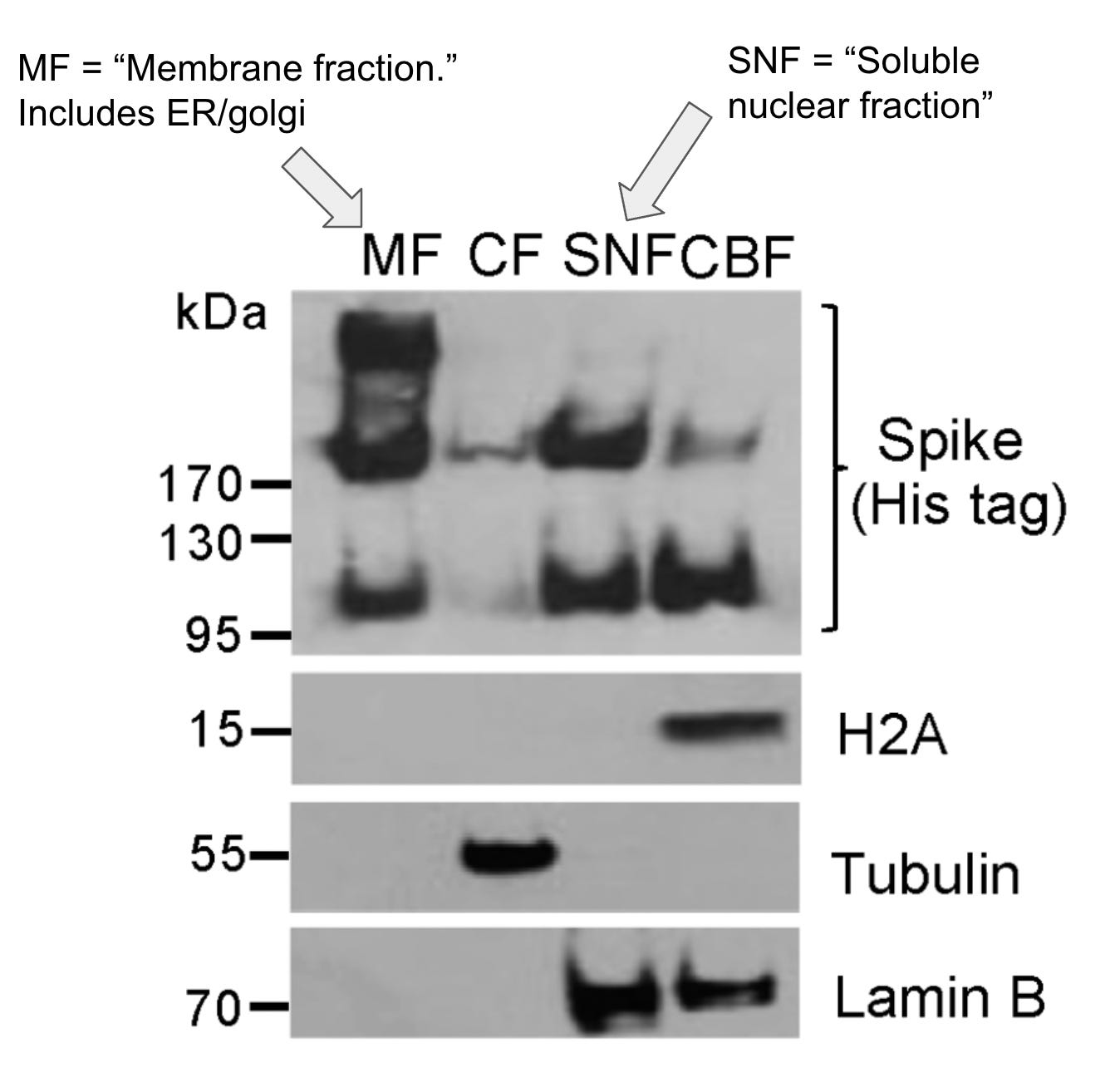
However, a caveat: this method does not perfectly separate out the cellular components. In the documents for the kit that was used by these researchers, they do mention that there can be contamination between fractions (highlighted in yellow):
They mention that the kit yields “sufficient purity” for “most” experiments studying protein localization but that’s just going to be based on the particular proteins this company tested on.
I’m inclined to believe these results, but again, it’s not a slam dunk.15
Using fusion proteins
The other study that used a slightly different method for determining subcellular localization was Study 3. In addition to using immunofluorescence, they got two different types of cell lines to express spike protein fused with “EGFP,” which is a fluorescent protein.
They therefore did not have to use primary or secondary antibodies in order to visualize spike protein, since this method produces a fluorescent spike. However, they still fixed and permeabilized cells in order to stain the DNA.
Using this method, as mentioned earlier, they did not find spike in the nucleus, which was also in agreement with their immunofluorescence results.
So what can we conclude?
So how do these results help us answer the question at hand? It’s hard to say, because there’s currently no basis to determine which of these methods is “better.”
They also used different cell lines and different transfection methods. The first study used human embryonic kidney cells. The second used cervical cells.16
I can imagine some people concluding from this that spike protein gets into the nuclei of kidney cells but not cervical cells, but again, we don’t know whether these results were just due to differences in the methods used.
Until we get better information, I think we should say it’s unclear whether spike gets into the nucleus of cells. My guess is that it could get into nuclei under certain scenarios, and perhaps only in certain types of cells, but I really don’t know.
Spike, nuclear abnormalities, and DNA damage repair
Whether spike protein gets into the nucleus or not, it can still affect the nucleus. And there is evidence that it does.
Study 7, which did not find that spike got into the nucleus of cells, still found that spike exposure led to nuclear fragmentation and other nuclear abnormalities in cells. The authors speculate it could be because the cells are dying or killing themselves.
Study 1 found that cells with spike exhibited lower levels of DNA damage repair.
From this, it might be tempting to think that spike directly acts on the proteins that repair DNA damage, within the nucleus. However, as far as we know, direct interaction between spike and these proteins has not been shown17, and it’s possible these effects could be indirect effects from spike.
For example, the effects on the nucleus might at least partially be a result of spike damaging mitochondria, which two studies found (here and here).
Damage to mitochondria releases reactive oxygen species (ROS) and pro-inflammatory cytokines (see here, here, here, and here).18
Both ROS and cytokines can have far-reaching effects on the cell, including effects on DNA and DNA damage repair proteins.
For example, see here or here for how ROS damages DNA, and here or here for how ROS affects DNA damage repair, including p53. And see here or here for how certain cytokines can lead to inhibition of DNA repair or DNA instability.
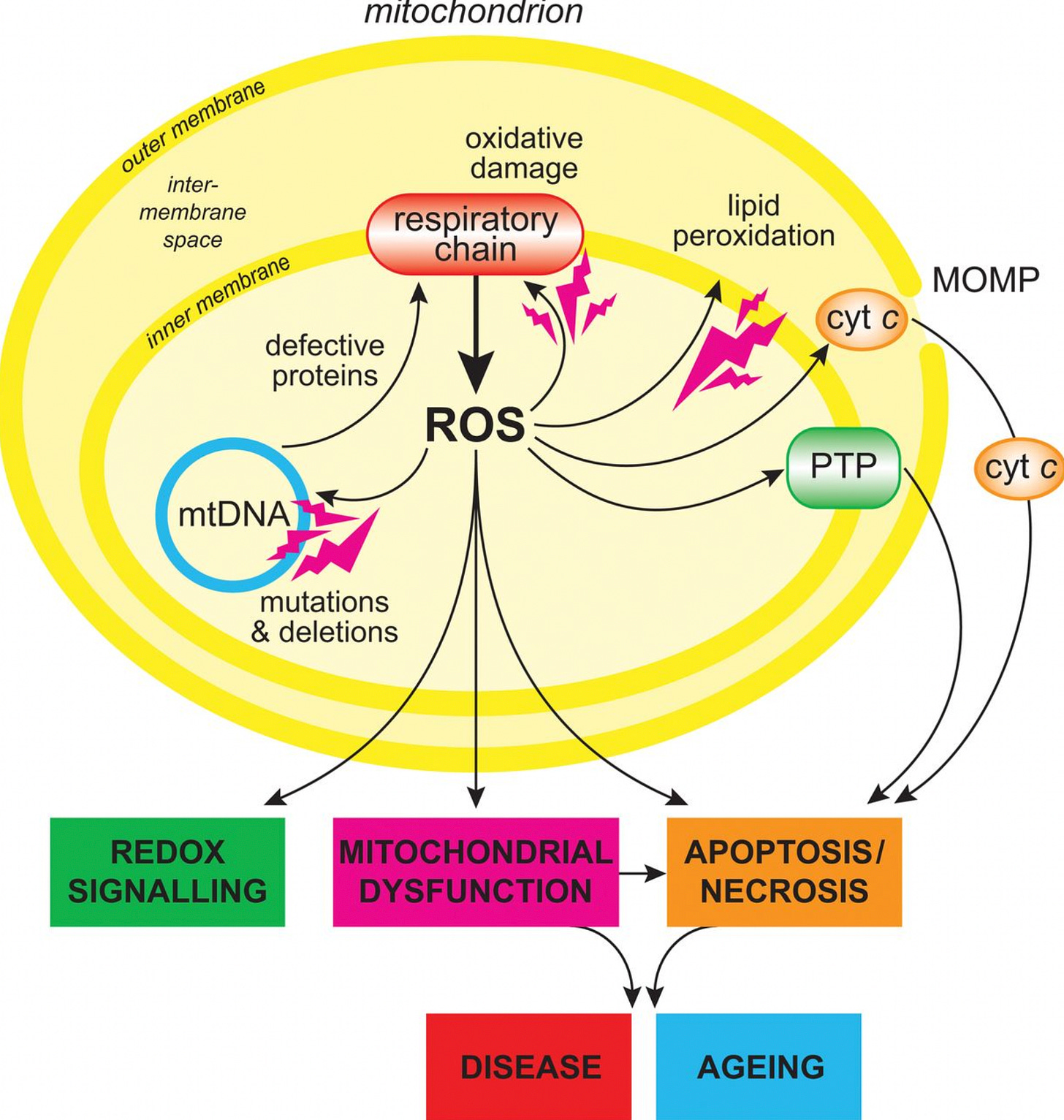
Then there’s the fact that spike expression can cause abnormalities in the endoplasmic reticulum (ER). According to this study, cells transfected to produce spike activated a process called Unfolded Protein Response, or UPR, which is indicative of ER stress.
This sends out all kinds of signals, including to the nucleus (see here and here).
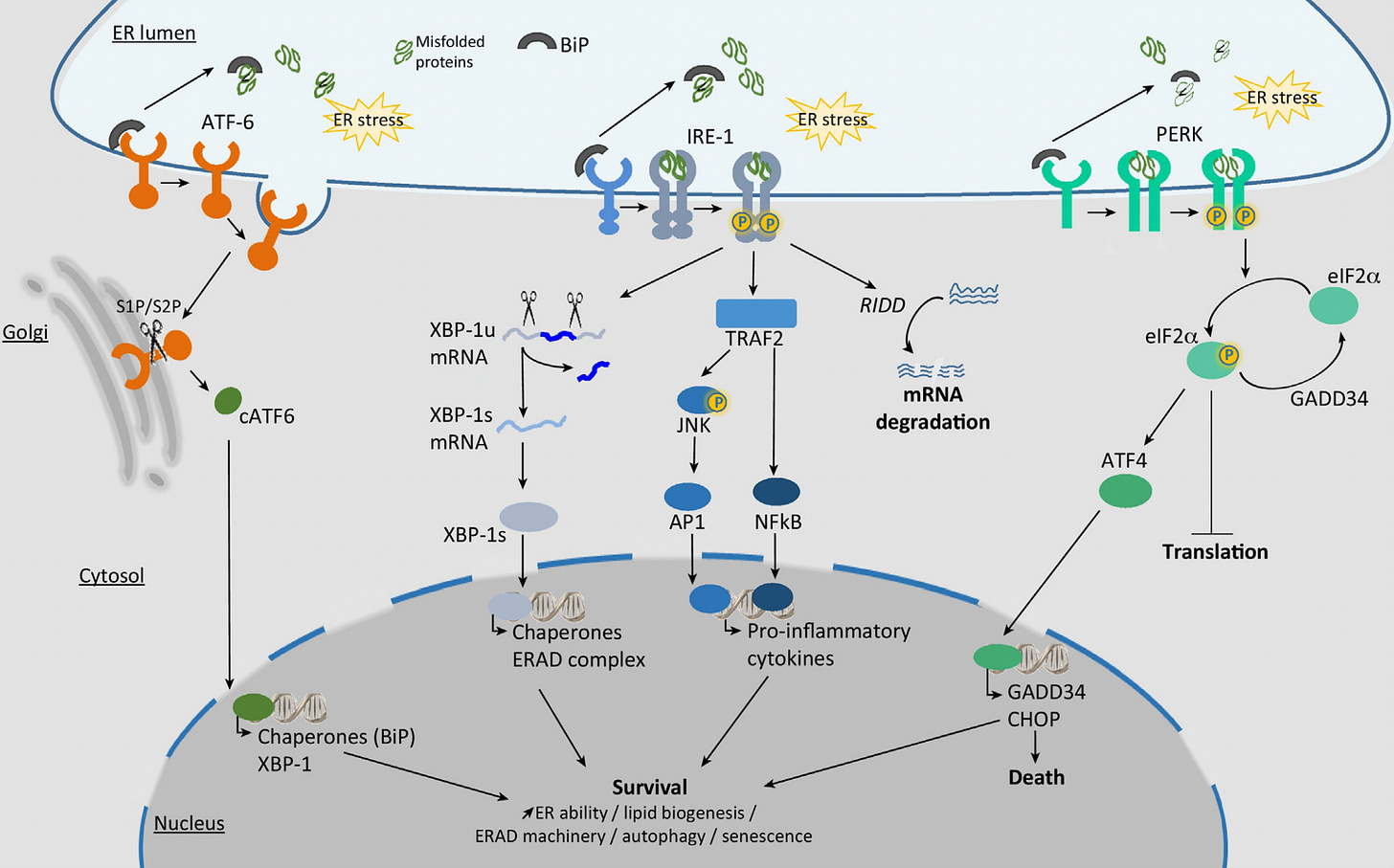
Given that we think spike protein first gets synthesized in the ER, it’s reasonable to guess that the dysfunction starts there, and radiates out to the mitochondria and nucleus, which are both in close association with the ER.
So spike seems to have far-reaching effects on the cell. Multiple organelles are affected by spike. That doesn’t mean that spike is directly acting on, or located inside all those organelles.
Embracing ambiguity
Some things are relatively straightforward to measure or interpret. For example, if you want to know how many mice will die from a certain medical intervention, you just have to count dead mice.
However, in molecular biology most things require all kinds of technology to measure. We need special tools to see or count things that are not visible to the naked eye: like DNA, RNA, proteins, modifications to proteins or DNA, etc.
Once you need a lot of technology to measure something, there are more opportunities for things to go awry. There will also be fewer people who understand the intricacies of the technology and know how to correctly interpret results.
For this reason, I’d wager that we’re not even close to aware of all the potential sources of artifacts out there. Many of our “findings” today will likely be shown to be erroneous someday.
This is why we should embrace ambiguity in science, and hold our beliefs lightly.
As I said in my last post, we should generally avoid saying things like, “The science is settled.” The reality of science is kind of a mess.19
Specifically, two proline mutations were introduced in order to lock or stabilize the spike protein into its prefusion conformation and make it more immunogenic, i.e., make it provoke more of an immune response.
This article focuses on whether the spike protein can get into the nucleus of cells under vaccination; what happens under viral infection might be different. Many viruses send some of their contents into the nucleus, and the way they do this varies; some viruses transiently disrupt the nuclear envelope, or gain access to the nucleus during mitosis, when the nuclear envelope is temporarily disassembled.
Other viruses have proteins that contain a nuclear localization signal, or NLS, which are short amino acid sequences that “tag” proteins for import into the nucleus. As far as we know, the SARS-CoV-2 spike protein does not contain an NLS, but there are a few other SARS-CoV-2 proteins that do (see Table 4 here).
Almost all eukaryotic cells have a nucleus but there are some exceptions, like mammalian red blood cells or sieve element cells in plants.
The part about the mRNA being expressed “transiently” is very misleading. For more on that, see this.
By the way, this paper later got retracted, but the data is still worth looking at. For what it’s worth, I don’t think this paper should have been retracted. In the retraction, some concerns had been raised about the use of the His-tag. It’s true that the use of His-tag can lead to artifacts; for example, antibodies used to bind to His-tag (the primary antibodies in this case) can sometimes bind to proteins that naturally occur in the nucleus. See here or here for example. But I think the authors of the paper had a reasonable response to this. The other thing brought up in the retraction was that the authors had made claims about “adaptive immunity” but the experiments performed had not related to adaptive immunity. In particular, the paper had made claims about V(D)J recombination, which as far as we know only occurs in lymphocytes (specifically B and T cells), and this study did not do any experiments with lymphocytes. So this is a fair criticism, but it’s more of an issue with the authors overreaching with their interpretations, not an issue with their data.
These two variants had different nucleotide sequences, but both encoded for the full length spike protein.
It’s even more complicated than I just mentioned. Even the product of what we get from the vaccine is not always consistent. According to this study, which sequenced spike protein that had been lingering in some people post-vaccination, occasionally people had mutant spike peptides. In other words, the sequence of the spike protein fragment was not what was intended from the mRNA vaccines. So it seems there are sometimes errors that happen when the vaccine mRNA is translated.
For example see this, which measured spike or S1 subunit of spike in the plasma of vaccinated individuals.
One way to do this is to produce fusion proteins where your protein of interest is fused to a fluorescent protein. This allows you to visualize your protein of interest without fixing and killing the cells. However there can be issues with this method as well (see here).
The authors of this paper claim that VP22 protein binds to the surface of cells which allows it to remain attached to cells during the washing steps before fixation. Then when cells are fixed with methanol, the VP22 rapidly gets taken into nuclei. When they used live cell methods to detect VP22, they did not find it getting into nuclei.
I can’t speak for the Pfizer/BioNTech studies however because they didn’t include their immunofluorescence protocols in their documents.
It’s not that I’ve done a comprehensive survey of researchers on this, but from everything I’ve seen it seems like researchers often use a particular protocol because someone in their lab told them to do it that way or they just did a google search or they found it in previous papers doing similar things. It’s rare they they will do some kind of comprehensive test to figure out the best methods. There are plenty of times when researchers follow a protocol not really knowing why they do it the way they do. I don’t blame them either; the amount to know in molecular biology is overwhelming.
There is one other thing that I’m not sure about with these results. You’ll see that they have some protein “controls” that are supposed to be proteins that are known to exclusively localize to the nucleus (lamin B or H2A) or cytosol (tubulin). Unfortunately they don’t include a control for the ER. Given that (1) a lot of spike was found in the membrane fraction that includes the ER, and (2) we don’t have a protein control for the ER here, we can’t say for certain that we don’t get some leakage between the ER and the nucleus in this assay, especially given how the ER and the nucleus are in close association in cells.
To add to the ambiguity: these cell lines are “immortal” and derived from cancerous cells, so they are not like normal cells in the body.
What I have seen related to this was an in silico (computer modeling) study that concluded that the S2 subunit of spike interacts with p53 and other proteins involved in DNA damage repair, like BRCA1/2. However, this was just a modeling study.
In fact the second study showing that spike caused damage to mitochondria also found an accompanying rise in ROS and pro-inflammatory cytokines.
I’m thinking I should have named my Substack “The mess of science” or something. It’s the theme that keeps emerging.
A lesson in living with astoundingly good writers on substack.
What an ordeal to write this all. Very impressive. I want to thank you wholeheartedly. Your articles are an absolute treat to read. Well laid out, detailed, nuanced reading for lay men like me.
One of your earlier articles about vaccin safety and how you use the term consilience was very powerful and should be read by many people. An update of that article would be very nice by the way. I would gladly read if things have changed for you.
This substack is as far as I am concerned essential reading. Hope it gets more coverage soon.
Your explanation of this uber-complex matter is wonderfully clear and the illustrations are helpful.
My impression is that the m-RNA vaccines are operating in ways that are still poorly understood. The systems that turn transcription on and off, when not interfered with, are astonishingly stable and self-correcting. The mechanisms are dynamic and as yet our investigative technologies are too clumsy to reveal their complexity.
Do you share my fear that Pharma is like Icarus and flying too close to the sun? It might be years, perhaps generations, before we can be sure of the safety of transcription therapies.